Why are smaller reactors attracting so much interest?
Small modular reactors (SMRs) are gaining increased attention as a major opportunity in clean power production. They are a welcome tool in the necessary transition from an energy system dominated by hydrocarbon combustion to one that produces more power for more people with dramatically reduced greenhouse gas emissions.
As a partner in Nucleation Capital, a venture capital fund that focuses on the commercial opportunities that are anticipated by advanced nuclear technologies and power systems, I thought it would be useful to discuss reasons why so many are eager for the arrival of the growing slate of smaller nuclear reactors that are under development. For important reasons there are few mentions of individual companies.
Like traditional large nuclear reactors, SMRs generate power from fission. They don’t produce any air pollution components like NOX, SOX, mercury or fly ash. Unlike large reactors, which were built as large infrastructure projects, the expectation is that SMRs can be constructed more quickly from prefabricated components so that they can be rolled out at an increasing rate once they have completed a necessary product demonstration phase.
Some energy observers see SMRs as the appropriate evolution of nuclear for use with a more distributed grid. Others believe the sector has been widely “hyped” in online energy forums but won’t be available in the time frame needed to address climate change. So how should those for whom the term SMR is just now entering their lexicon think about this technology?
Why does fission excite anyone?
Uranium and thorium are two of nature’s most incredible clean energy storage assets. If completely fissioned, a handful of nuclear fuel weighing a kilogram contains more stored energy than 50 large tanker trucks filled with petroleum.
At the current diesel fuel price of $5.60 per gallon, 50 trucks can carry more than $3,000,000 worth of fuel. In contrast, nuclear power plant owners pay approximately $1,700 per kilogram of fuel in the form of finished assemblies.
The tiny waste production per unit energy released is an inherent aspect of concentrated fission reactions. Unlike combustion, all ingredients needed for fission are contained inside fission fuels. (Combustion needs an external source of oxygen in greater masses than the fuel itself.) The mass of fission wastes is slightly less than the mass of fission fuel; the mass of combustion wastes are about 2.5 times the mass of input fuel.
No fission product wastes need to be routinely removed to allow the reaction to continue operating for its design fuel cycle. None need to be discharged to the environment. Fission reactors are clean enough, safe enough and independent enough to operate inside sealed submarines carrying crews of several dozen people. Those submarines have gone to every part of every ocean on the planet.
Fission even works in the vacuum of deep space.
Those physical and economic facts almost beg power plant designers to think about building a wide variety of machines in order to use that amazing source of energy in as many parts of the diverse global energy markets as possible. Power systems using combustion fuels range in size from model trains to multi GWe power stations. Fission-based power systems need sufficient size to support a chain reaction, and to provide adequate shielding, but that still leaves a wide spectrum of potential applications and sizes.
Today, advanced nuclear innovators are designing reactors that will meet the needs of a much broader range of energy users than traditional nuclear could previously address.
Why did fission reactor unit size get so large?
The earliest reactors were small; the core of EBR-I (Experimental Breeder Reactor I) was roughly the size of cylinder that could snuggly contain an American football. It produced a 1.5 MW of heat and 200 kW of electricity. That was enough to power the building that housed it.
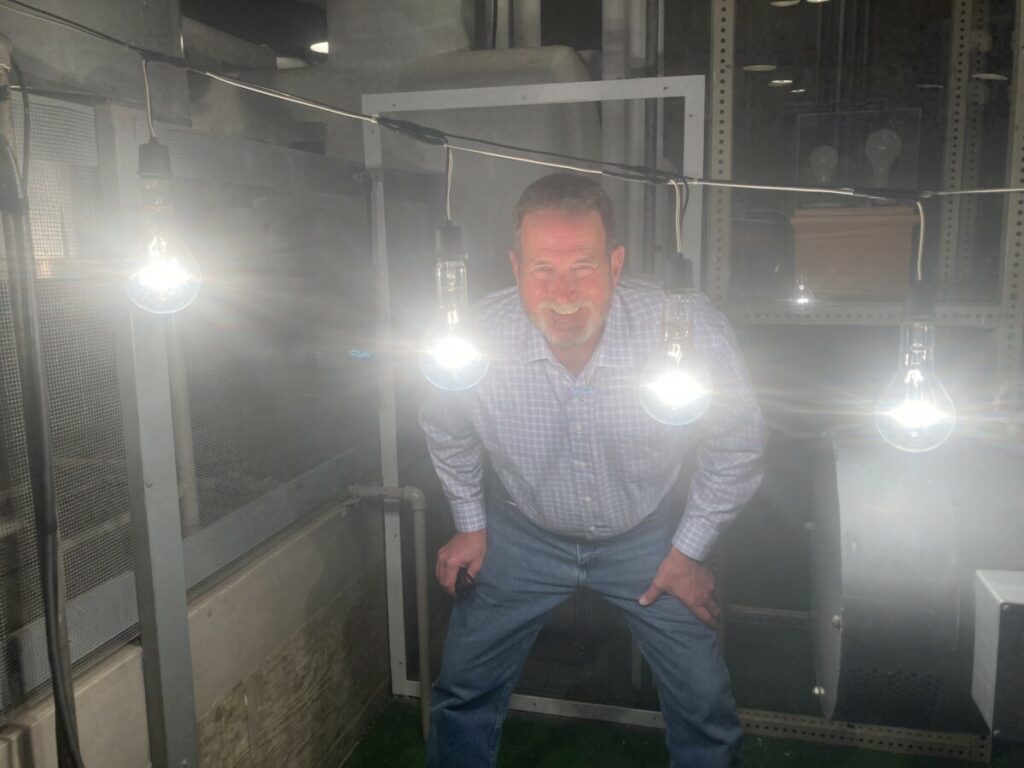
But since the dawn of the First Atomic Age, the primary design trend has been to strive for ever larger units in hopes of reducing the cost of the electricity they generate. In the 1950s, 60s and 70s, engineering degree programs taught that the “economy of scale” meant that equipment cost did not grow as rapidly as capacity.
For example, a pump that could produce ten times as much flow should only cost four times as much based on cost computations using input materials.
Design engineers learned by experience that bigger factories, bigger refineries and bigger mines could produce cheaper commodity products.
Seeing nuclear power plants as electricity factories, they could not help but believe that achieving economy of scale required them to design ever larger reactors and to place them in increasingly large groups at massive power stations.
There is a diseconomy of scale with super-sized units

Larger units can successfully use the economy of scale to lower the cost per unit of output but it isn’t the only kind of scale that can drive down costs. Ever larger units can also run into diseconomies of scale that plague mega-projects in construction, mass transit, sports complexes, and airports.
The experience of the industry in building the Vogtle AP1000s shows that there is such a thing as too large. In contrast, the economies of scale that we believe will aid in the appeal of SMRs takes the form of mass production and is expected to enable the construction of SMRs to more closely follow the declining cost curves experienced by wind and solar projects.
How can smaller reactors be produced and operated economically?
After observing the challenges associated with building only very large nuclear plants, there is a growing field of nuclear system developers who consider nuclear power plants to be a product, not a factory. A phrase that is repeatedly heard at gatherings of modern nuclear system developers is “We want to build airplanes, not airports.”
Entrepreneurial companies that see nuclear power systems as products understand that “scale” means building large quantities of the same product. They need to be positioned to meet the needs of a sufficient number of customers who want to buy enough machines to provide the opportunity to capture cost reductions from “experience curves” where cost declines as a result of cumulative product volume.
One advantage of smaller systems is the improved ability to use factory manufacturing techniques. Of course, the components used in conventional large reactors are produced in factories, but then they are individually shipped to the site to be assembled into an operating plant. With reactors that have the size and complexity closer to that of large ships or commercial aircraft, it is possible to assemble and transport complete or nearly complete products.
Factory workforces have many advantages over site construction workforces. They can improve productivity by repeating similar tasks regularly, They can live and work in cities served by mass transit. They can implement quality assurance techniques and environmental consistence systems that are difficult to achieve at remote large plant assembly sites.
Several of the entrepreneurial advanced nuclear companies are looking to scale their businesses using a hybrid approach. They recognize the need to build large quantities of their power- and heat-generating machines but they have found that there’s a limited universe of customers who want to operate nuclear power systems under current and foreseeable oversight regimes.
One solution to this problem is “Energy as a Service.” Companies can build, own and operate a fleet of their own small modular reactors to produce electricity and heat as the products they sell to willing customers. At least some of the companies following this path are keenly aware that there are numerous advantages to co-locating a significant number of the members of their nuclear fission fleets on each site they develop.
That statement is especially true under current regulatory requirements for security, oversight and quality assurance. Large numbers of smaller units on a single site also take advantage of repeatable, consistent work for the inevitable site-specific parts of erecting a power station. They can share transmission and cooling infrastructure, training, emergency response and administrative facilities.
One more variation on the SMR business model is the detectable emergence of developers that want to build on skills they’ve gained in deploying, owning and operating other kinds of power systems. They believe that certain SMRs, once designs are approved, can be readily integrated by project developers that specialize in engineering, permitting, construction, local politics, working with regional operators, and developing logistical supply chains for projects that blend clean energy production for local grids with water purification, carbon capture, hydrogen production and other revenue opportunities.
What about the waste for small modular reactors?
Lindsay Krall, Rodney Ewing and Allison Macfarlane recently published a paper titled Nuclear Waste from Small Reactors in the Proceedings of the National Academies of Science describing the larger surface to volume ratio of smaller reactors as a disadvantage.
Aside: Neutron Bytes published an article with extensive commentary on the study; though there is some overlap between that article and this one, this contains a few additional points of interest. End Aside.
The paper authors chose to conduct their study using publicly available information on three (out of dozens) small modular reactor designs. They looked at an early version of the NuScale Power Module, Terrestrial Energy’s IMSR™, and Toshiba’s 4S (a reactor system that has not been actively marketed since 2011.)
To the surprise of the lead author, that paper received unusual attention from the press.
I didn’t really know how the article would be released. There was a copy of the paper circulated to the media or to the press some five days in advance of the article’s publication. So, reactor developers were contacted by the press about the article before it was even published. As a scientist, I was just thinking, “Oh, thank God, this paper got accepted, and I don’t have to work with it anymore.” But then the release of the paper shocked me.
Diaz-Maurin,François Interview: Small modular reactors get a reality check about their waste, Bulletin of the Atomic Scientists, Jun 17, 2022
The attention was likely related to press releases issued by the host institutions before the study was actually published.
Both NuScale and Terrestrial Energy challenged the study’s conclusions about their systems. Dr. Jose Reyes, NuScale CTO, wondered about the way advanced copies of the study were released to the press and asked PNAS editor-in-chief May R. Berenbaum if PNAS had implemented a new policy about paper promotion.
It seems likely that summaries of the paper’s tenuous conclusions will be repeatedly introduced into discussions of small modular reactors. If not fully understood, the assertions about increased waste production might hamper SMR deployment.
The paper stated that smaller cores leak a larger portion of the fission neutrons and those neutrons activate structural materials. It also stated that increased neutron leakage leads to lower fuel efficiency because a reactor with increased neutron leakage needs a higher concentration of fissile material to maintain criticality.
Discussions with several nuclear design engineers that are not working on SMRs confirmed that those statements contained some truth and were worth consideration, but also described how they did not tell the complete story about waste generation from smaller nuclear plants.
Those design engineers pointed out that a marginal increase in the amount of waste generated per unit energy output would not make much of a difference in the effort needed to address long term storage or disposal of radioactive materials.
The paper expressly ignored the potential to reduce radioactive waste by recycling material and fuel.
This study also neglects to consider reprocessing, recycling, and dilution because these treatments will not eliminate the need for the storage, transportation, treatment, and disposal of radioactive materials.
Krall, L.M.; Macfarlane A.M.; Ewing, R. C. “Nuclear waste from small modular reactors” PNAS Vol. 119, No. 23 May 31, 2022
In her interview with the Bulletin of the Atomic Scientists, Dr. Krall pushed back on the headlines that emphasized the high end of the range of estimates offered. She said that high end estimate of increased waste generation only applied to sodium, a coolant proposed for use by several small and advanced reactor developers.
…for a sodium-cooled reactor, for instance, that sodium coolant is likely to become low-level waste at the end of the reactor’s lifetime, because it becomes contaminated and activated during reactor operation. So, the “up to 30 times more waste” that’s been driving the headlines, it’s mostly the sodium coolant.
Diaz-Maurin,François Interview: Small modular reactors get a reality check about their waste, Bulletin of the Atomic Scientists, Jun 17, 2022
For sodium waste, reuse in new reactors has the potential for a dramatic decrease in waste that needs to be processed for disposal, but only if there is a growing population of sodium-cooled reactors.
The potential for reuse of sodium from reactors is limited. The most likely utilization would be as a reactor coolant, but there are currently no LMFRs being constructed where sodium waste is available. (Emphasis added)
IAEA Radioactive Sodium Waste Treatment and Conditioning, Jan 2007. p. 46
Small reactors also have some advantages in fuel and nuclear plant material systems that include recycling. They are going to be easier to disassemble for some of the same reasons that they will be easier to assemble. Recycling factories can be less costly when designed to handle a more steady flow of smaller pieces and parts.
The Krall et. al. study also did not credit advanced reactors with fuel efficiency gains that arise from using operating temperatures that are significantly higher than those that are possible in large light water reactors.
Few of the teams designing SMRs point to reductions in waste generation as a major selling point, but quite a few of the designers point out the potential that fast reactors have for using recycled materials from the current used nuclear fuel stockpile and describe the efficiency gains from reactor systems that are capable of achieving much higher temperatures than conventional light water reactors.
Engineering is a profession that recognizes tradeoffs. Improvements in one design measure often results in a reduced performance in another design measure. Establishing priorities and determining the best overall choices requires expert, detailed knowledge that often isn’t available to outside academics. Small modular reactor developers and their backers have evidently determined that improving passive heat removal, constructibility, financial performance and customer acceptance are more important than the potential for a marginal increase in waste generation.
Waste from smaller modular reactors needs to be well managed, but neither the waste nor the misleading media headlines that appear to detract from the clear benefits of this emerging technology, should be seen as significant hurdles to their successful development.
This is a great article on the status of small reactors. As a retired nuclear engineer who has worked in a variety of roles including at a nuclear utility, regulator, and consulting company, I am supportive of the move towards small modular reactors. I agree with the many points raised by Mr. Adams. Let me share some insights that I have observed through the decades. On the Diseconomy of Scale issue: decades ago as a graduate student, my class undertook an exercise on the very topic. Yes, it is true that the overnight cost in terms of dollars per kilowatt of installed capacity decreases as the reactor size increases. But we showed that, at high borrowing costs during construction and commissioning, the compounded interest during construction of large reactors could more than offset this economy especially as construction time stretches out the commissioning date. On the other hand, operations and maintenance costs, normalized for unit size and power generation, can at some point overwhelm all other costs including fuel and depreciation. It is no coincidence that the first units to permanently shut down have been the smaller, single unit sites where labor costs have been relatively high — a fixed O&M budget divided by too few megawatt-hours produced will come back to hurt the economics at some point (think of the increasing challenges facing Yankee Rowe at 186 MWe, although that unit was primarily shut down at the time because of reactor pressure vessel embrittlement uncertainty issues). Take one particular area, security, as an example. Without getting into the specifics of safeguards information, a situation where at current commercial reactors the number of security personnel can be greater than the operations staff is not a business model that could be successfully carried over to the fleet of smaller reactors. This is also the case for emergency planning cost. Siting is yet another matter: easier to license and construct fewer large reactor units on a moderate number of sites than thousands of units on hundreds of sites distributed around the country. Each site can be its own lure for obstructionism. A paradigm shift is necessary if small, modular reactors are to become the predominant business model, I believe.
Donald:
You are correct in stating that we need to have a paradigm shift to ensure small modular reactor success. We need to push for answers and successes that keep the already shifting paradigm on track.
With security, there are solid answers being developed. Many of the SMR projects I’ve been reviewing take the challenge seriously and have incorporated security by design in ways that will seriously reduce the required security manpower. Unlike conventional reactors that were built many years before the increased ratcheting requirements in force today, modern power plant designs protect critical areas and provide clear sight lines. They incorporate modern security monitoring systems with sensors and cameras….
They’re also working on changing security paradigms by explaining how a large, heavily armed guard force capable of withstanding force on force drills can add more vulnerability than it removes.
Site licensing will also need to change. Generic environmental impact assessments for small reactors would help. Replacing coal plants with reactors should be given the credit it deserves as an improvement to the local environment, for example.
There’s lots of work to do. I hope you find the time to continue making a positive contribution to the discussion.
Its also easier for small reactors to be deployed on floating barges for the offshore production of electricity and desalinated water and even the remote ocean production of synthetic fertilizers (ammonia) and fuel (methanol, gasoline, and jet fuel).
Methanol can be used to replace marine fuel for ships modified to use methanol. Methanol can also replace natural gas in natural gas electric power plants cheaply modified to use methanol. In both systems, the CO2 can be captured and recycled to produce more methanol through nuclear energy.
Thank you Rod for your clear thinking and ability to express it.
Wow that is really a problem. We might have 2 football fields of slightly used uranium. Show me a problem that actually kills some folks.
The reason I am excited about SMR’s especially “micro” reactors is that they would scatter a bunch of reactors all over the place. This would increase their acceptance greatly! We need thousands of reactors of all sizes to power a fully electric grid and potentially produce syn fuels. If small reactors get started we will be looking at hundreds of them in fairly short order. A product can be improved a project can only be completed.
Some of the reactors will be working in the fast breeder range using the left over actinides the thermal reactors will be producing.
It would be terrible to have two football fields of breeder fuel.
What would encourage the construction of small modular reactors?
What caused the closure of many reactors in the United States?
It was natural gas.
It seems not that long ago that there was to be a nuclear renaissance. Natural gas prices were high and there was a buzz about more nuclear plants.
Then the fracking boom hit and we were promised ample natural gas for the next 50 years. Natural gas was cheap. Coal plants began to close like falling dominoes. Nuclear plants followed the closures with closures of their own. Combined cycle natural gas plants offered cleaner operation with a far smaller operating crew for the same megawatt output. These were built all over the US. This raised the demand for natural gas. American industry also used the abundant natural gas for various productive activities. Natural gas lowered the greenhouse gas emissions for the US utility industry. Life was good.
Now it is 2022 and perhaps we are seeing the beginnings of the end of the natural gas party. Gas prices have been rising. Some gas is being sold to Europe as LNG to make up for Russian gas losses. Can we expect these prices to fall soon? See EIA link.
https://www.eia.gov/todayinenergy/detail.php?id=52698
Could the rising price of natural gas cause an attempt to actually build some of these small modular reactors instead of just talking about them?
In addition to this even conservatives have shifted their stance on global warming in recent years to grudgingly accept it as reality.
Many of Donald’s concerns, about costs of security, siting, and emergency planning, could be addressed or alleviated by placing multiple SMR units on a single site. That’s what NuScale is doing, at UAMPS, etc..
Also, with respect to emergency planning, isn’t it the case that many SMRs have a much smaller EPZ, perhaps even the plant site boundary? The much lower potential release for SMRs should reduce EP costs. My understanding was that NRC is receptive to greatly reduced EPZs.
Eino:
I’d add something else to your list of pressures pushing natural gas demand (and prices) up. Our first LNG export shipment took place in 2015. US has moved rapidly to top of leader board among LNG exporters. When all facilities are running, we export about 11 bcf/day. That’s ~10% of our production leaving US shores each day.
Much higher prices in international market. I don’t begrudge suppliers who are seeking most profitable markets.
Thanks Rod for the excellent rebuttal of the arguments made
by Krall et. al. in their paper against SMR designs, especially
their explicit ignorance of potential advantages made possible
by recycling and reuse, as they have said, “because these treatments
will not eliminate the need for the storage, transportation, treatment,
and disposal of radioactive materials.”.
Perhaps Krall et. al. have not heard about Deep Isolation’s approach
to nuclear waste disposal using deep borehole technology, which
seems quite capable of addressing all of the issues they raised.
Perhaps Krall et. al. have also ignored the effects of reprocessing
and storing highly radioactive fission products separately from
reusable fuels. Those effects include the reduction of required
storage lifetimes of fission products by 3 orders of magnitude,
which can greatly reduce the complexity of deep borehole siting
requirements.
Licensing seems to be the biggest obstacle right now, particularly when it comes to Gen IV designs, which is big part of why virtually all orders for new reactors globally are for LWR’s. One need look no further than the CRBR site, now proposed to be the site for a BWRX-300. Probably the saddest commentary anywhere on the complete and utter failure to move beyond the LWR. You also see it in the UK where AGR’s are being replaced with PWR’s. Technological regression on this scale will inevitably result in the fission industry’s inability to compete long term. The ~33% thermal efficiency and sub-5% fuel utilization characteristic of LWR’s will all but guarantee it.
AGRs get miserable fuel utilization since the clad is stainless steel and achieve about 37% of PWR discharge burnup. That is not to say that CO2 cooled systems have no future, but AGRs did not pan out to be competitive with the pwr and that’s why the design did not proliferate globally. I’m a fan of the concept, and I believe there is a Gen 4 in there somewhere, but it is not in the running. None of the Gen 4 are a leap in operability or cost; all things considered (i.e. fuel handling, gaseous waste, etc. for MSR, SFR) not a safe bet for a sure investment…. Thus PWRs are built in the developing world.
The AGR unique selling point was online refuelling and hence higher availability (I realise other reactors like CANDU can be refuelled online but PWRs can’t). The higher availability didn’t work out as PWRs got much slicker at refuelling. Add the larger plant required for single phase gas as a primary coolant, larger if lower pressure reactor chambers and larger overall buildings and AGRs lost.
I imagine the desire to power Britain with British technology was among the main selling points for the AGR; additionally, the local talent probably had desire/direction to recoup/build-upon some MAGNOX sunk cost as well. Most power reactor concepts other than the LWRs have minimal excess reactivity and require frequent or constant fuel handling. The tremendous excess reactivity of LWRs at < 5% enrichment is a virtue of these reactors semantically similar to "energy storage" – it has few downsides (mostly in analytical accident scenarios). Those who extoll the virtues of Gen4 could better understand how LWRs are the benchmark, setting the bar quite high for ease/cost of operations, safety, reliability, waste volume, etc.. They are no more 'dinosaurs' than commercial airliners, which share the general architecture of military airplanes developed prior to 1950. We don't see widespread criticism of airplane/automobile manufacturers for failing to reimagine their incrementally improved designs…, but ‘futurists’ are quick to criticize the LWRs. The appeal to novelty fallacy (e.g. SMRs, Gen4) adds another confounding distraction in a public discussion that hasn't sufficiently ripened; we haven’t had that “pain at the meter” to spur new construction. Perhaps successive generations will tolerate a slowly reduced standard of living, reserving pain for those who once kept their homes at 20°C and sipped plastic straws… there could be ANOTHER new normal where pro nuclear folks (like myself) remain frustrated.
Factory-based assembly and disassembly of SMRs would seem to provide an opportunity for
closed fuel cycles based on used fuel reprocessing, depending on the regulatory status
of reprocessing. Recently I’ve heard in a webcast talk (given by Zabrina Johal of General
Atomics) that reprocessing is forbidden in the US due to “weapons proliferation concerns”.
What is the current US regulatory status of reprocessing? Would reprocessing of used
fuel in an SMR factory site be forbidden by current regulations even though recycled fuel
is only used in the vendor’s reactors? If so, would US Government action (e.g., by the DOE)
be needed to overcome the prohibition in order to permit reprocessing in SMRs?
Zabrina Johal of General Atomics is also a board member of the National Museum
of Nuclear Science and History in Albuquerque NM, which will be the venue in Oct 2022
of the 11th Thorium Energy Alliance Conference (TEAC 11). I’ve sent the museum a
question that I hope will be forwarded to Ms. Johal, asking for the details of the
“weapons proliferation concerns”.
AFAICT the concerns may be related to transportation or trading of MOX fuels which
conceivably might raise concerns about weapons proliferation. But concerns about
weapons proliferation raised by secure in-house reprocessing as part of factory based
SMR assembly and disassembly? Not so much. AFAICT.
If I haven’t received a reply from either Ms. Johal or someone on the staff of the National
Museum, perhaps Rod might want to try to contact her directly at TEAC 11 where he is
listed as an expected attendee.
Based on her talk, Ms Johal’s company is an all-in supporter of nuclear fusion (thermally
hot variety). Perhaps Rod might also want to ask her how long she expects the transition
to take from ITER ignition (millions of degrees Celsius for a few msec) to 24x7x52 reliable
clean energy at scales comparable to those projected for fission-based SMRs. 🙂
When we find that Krall et al. use the loaded word, “waste” 99 times, and the aggressively loaded term, “spent fuel”, “SNF” 110 times, it is time to check where these guys are coming from. All three authors are members of the “Center for International Security and Cooperation”, whose website declares that “Since the beginning, one of our key goal [sic] has been to reduce nuclear risk…” They seem to believe that they could stamp out nuclear war by obstructing fuel reprocessing in the US. To be wary of giving credence to such voices, we should avoid using the word “waste” and never speak of fuel as “spent”.
Ironically, they point to Toshiba’s 4S , which can be configured as a plutonium burner, itself a solution to their fear of ever-accumulating “nuclear waste”.
Actually I’m a fan of the concept of AGRs and one of my favorite advanced reactor designs is an AGR, a micro-modular reactor (MMR) design by Ultra Safe Nuclear Corp (https://usnc.com). It’s helium cooled, TRISO fueled, and seems designed especially well for remote communities and mining sites (think coal-mining replacement). Among other things, it avoids corrosion issues associated with molten salt fueled reactors. Not sure how they deal with reactivity swings associated with xenon-135 generation.
Like other SMRs, they have passive safety, factory-based assembly and disassembly, and unlike others, they’re using 3D printing to lower fuel manufacturing costs.They’ve just opened a fuel-manufacturing site this month in Oak Ridge, Tennessee.
The main limitation I see is that their TRISO fuel design makes reprocessing even more expensive. But if reprocessing is off limits in USA due to old
NRC regulations intended to block manufacture and trading of MOX fuels, it might make sense to consider closed open-pit coal mines as deep borehole sites for used fuel disposal.
What do you think?
Scaryjello,
I should have added the context to the above comment that it
was all in response to your previous largely positive comment on
AGRs.
[I assumed that since I initiated the comment using the ‘Reply’
button that my response would be placed in-line with yours.]